|
Concealing coloration allows prey animals to blend into the background, making them
harder for visual predators to detect (reviewed in
Bond 2007,
Diamond & Bond 2013).
We intuitively tend to think of concealing coloration in terms of our own perceptions:
How well does a color pattern correspond to an animal's surroundings?
How hard is it for a human observer to find the animal? But visual search is actually
a very complex process. In addition to the resemblance between the animal and its
background, prey detection is strongly dependent on observation conditions, on
characteristics of the predator’s visual system, and on short-term changes in the predator’s
focus of attention and searching speed. To reduce ambiguity, Endler (1991) defined a
cryptic color pattern as one that resembles a random statistical sample of the background.
He proposed to sample patches of color from both the background and the animal, determine
the multivariate distributions of hue, saturation, and brightness in each, and then measure
crypticity in terms of the correspondence between the two sample distributions.
Endler's definition was primarily in terms of color physics, but crypticity is also
affected by differences in spatial frequency, in the mean size and variability of distinctive color
patches. The impact of even simple differences in spatial frequency is shown below
with a set of six artificial moths (A) on several different background
configurations. The intensity histogram of the background in B corresponds
closely to that of the moths, but the average spatial frequency is somewhat higher (i.e.
background patches are smaller than moth patches). The distinctive local spatial
correlations in the moths draw the viewer’s attention, making a contrast to the uncorrelated
texture of the background. In C, the background pixels were drawn from
independent light and dark intensity distributions that minimally overlapped those of the
moths, but the average spatial frequency was reduced (i.e. larger patches, comparable to
those on the moths). In this treatment, the moths are less cryptic, in terms of the distribution
of gray-scale colors, than the moths in B, but both humans and blue jays
find them far harder to detect. In D, both the intensity and the texture
distributions of the background match those of the moths. And the moths essentially
disappear.
There are also effects of the background pattern that are the result of aspects of visual
cognition. A background that is intricate and complex, containing many contrasting elements
over a range of spatial frequencies, can require a prolonged and careful search, even if the
prey are not otherwise particularly cryptic
(Bond & Kamil 2006,
Bond 2007).
This is the basis for the visual puzzles in many children’s books (e.g.
Where's Waldo; Handford 1987), in which the task is to locate a particular item
in a highly detailed and cluttered image. There may be only a limited resemblance between
the item and the distracting elements of the background, but because there is so much
information to be processed, the search can be very laborious
(Diamond & Bond 2013). We have addressed a variety of aspects
of visual search and detection of concealingly colored animals using blue jays
(Cyanocitta cristata) searching computer displays for images of cryptic moths.
Underwing moths are one of the most familiar examples of cryptic coloration. They
sit motionless on tree trunks during the daytime with their forewings closed
over their backs. The color patterns of the forewings resemble the surrounding bark,
making them very difficult to detect. An unusual feature of cryptic insects in
general, and of forest moths in particular, is that they are often polymorphic --
the individual species occur in multiple, visually distinctive forms.
In underwing moths, the polymorphism is mainly in the cryptically colored forewings;
the hindwings, which are concealed from view when the insect is at rest, are generally
uniform within species.
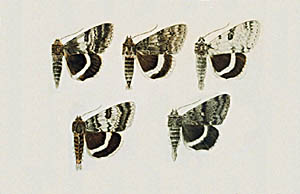 |
Five forms (or "morphs") of the Relict Underwing Moth,
Catocala relicta. (Revised from Barnes & McDunnough
1918). Note the variable forewings and the relatively uniform
hindwings. |
In North America, blue jays frequently
prey on these woodland moths during the daytime. They can pick them off even when these cryptic insects are resting motionless on tree trunks. In the 70s and 80s, Kamil
and his students found that jays in the laboratory showed these same impressive
detection abilities when the birds were required to locate cryptic moths in slide images
(Pietrewicz & Kamil 1977;
Pietrewicz & Kamil 1979).
We converted this natural predator/prey system into one that could be digitally manipulated,
reducing the resolution of gray-scale images of real cryptic moths to 16x16 pixel icons that
we overlaid on textured, fractal backgrounds. The backgrounds were reverse-engineered
from the moth images, allowing us to produce a broad range of task difficulties.
|
Five
digital moths displayed on a uniform gray background (top)
and on three different levels of cryptic background. With
practice, most blue jays have little difficulty detecting
moths even at Level 6, though their performance declines
at higher crypticities. Jays can only rarely find moths
at levels of 8 or 9. |
We
presented the moths, one at a time, to the birds in an operant chamber.
In each trial there either was or was not one moth image imbedded in
one of the fields of cryptic background on a computer monitor (see
below). If the bird found a moth and pecked it, the peck was registered
by an infra-red touch screen, and the bird was rewarded with a food
pellet in the central well below the perch. If the bird did not find
a moth, it pecked the green circle, in which case the next trial began
immediately. The bird was never informed if it overlooked a moth, and
if it pecked an area of background with no moth present, the time to
the next trial was substantially delayed. This discouraged random
responding.
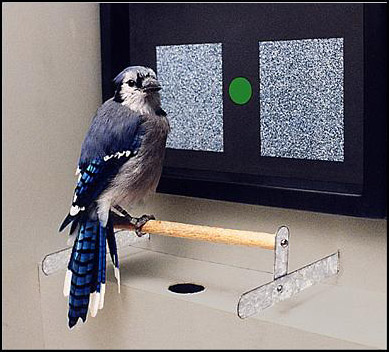
Our initial experiment
(Bond & Kamil 1999)
validated the technique, showing that it produced effects similar to
earlier studies using photographic images. We found that the birds were more
accurate at finding moths after a series of trials of the same type of prey than
during random presentations of differing prey types. We also found
interference effects, in which inducing a jay to search for one type
of moth reduced the likelihood of its finding an alternative
type.
References from Other Sources
Endler, J.A. (1991). Interactions between predators and prey. In
Behavioral Ecology, J.R. Krebs & N.B. Davies (eds.), pp. 169–96. London:
Blackwell.
Handford, M. (1987). Where’s Waldo? Boston: Little, Brown.
|